
국내외 수전해 기술 및 대규모 실증 프로젝트 진행 현황
2024 The Korean Hydrogen and New Energy Society. All rights reserved
Abstract
Global efforts continue with the goal of transition to a “carbon neutral (net zero)” society with zero carbon emissions by 2050. For this purpose, the technology of water electrolysis is being developed, which can store electricity generated from renewable energies in large quantities and over a long period of time as hydrogen. Recently, various research and large-scale projects on ‘green hydrogen’, which has no carbon emissions, are being conducted. In this paper, a comparison of water electrolysis technologies was carried out and, based on data provided by the International Energy Agency (IEA), large-scale water electrolysis demonstration projects were analyzed by classifying them by technology, power supply, country and end user. It is expected that through the analysis of large-scale water electrolysis demonstration projects, research directions and road maps can be provided for the development/implementation of commercial projects in the future.
Keywords:
Net-zero, Power-to-gas, Renewable energy, Water electrolysis, Green hydrogen키워드:
탄소중립, 재생 에너지, 수전해, 그린수소1. 서 론
2050년까지 탄소중립(net-zero) 사회로의 전환을 목표로 전 세계적인 노력이 계속되고 있다. 이에 화석연료 기반의 전력 생산 기술에서 벗어나 재생에너지를 이용한 전력 생산 기술이 연구 개발되고 있으며, 실증 프로젝트 또한 활발히 진행되고 있다. 재생전력 생산을 위한 대표적인 재생에너지로는 태양광에너지와 풍력에너지를 들 수 있다. 태양광에너지 및 풍력에너지를 통해 생산한 재생전력은 생산 과정에서 이산화탄소가 발생되지 않아 친환경적이며 탄소중립 실현에 주요한 에너지이다.
하지만 재생전력은 출력의 변동성으로 인해 불안정한 전력 공급, 기존 전력 계통 연계의 불확실성 등의 한계점이 존재한다. 이러한 한계점으로 인해 재생전력의 기존 전력 계통으로의 공급 과잉을 막기 위한 출력 제한(curtailment)이 발생하는 상황이다.
위와 같은 한계점들을 보완하기 위해 전기에너지를 화학에너지로 대용량, 장기간 저장할 수 있는 power-to-gas (P2G) 기술이 부각되고 있으며1,2) 대표적인 P2G 기술로는 수전해(water electrolysis) 기술이 있다3,4).
수전해 기술은 전기(e-)를 공급하여 물(H2O)을 수소(H2)와 산소(O2)로 분해하는 전기화학 반응을 이용한다. 결과적으로 전기에너지인 재생전력(e-)을 화학에너지인 수소(H2)로 저장하는 기술이다. 위의 전기화학 반응을 통한 에너지 저장 전 과정에서 이산화탄소의 배출 없이 생산된 수소를 그린수소(green hydrogen)라 한다. 최근 전 세계적인 탄소중립 기조에 발맞춰, 탄소 배출이 없고 에너지를 효율적으로 저장할 수 있는 그린수소 생산을 위해 연구실 및 실증 프로젝트 규모로 수전해 기술에 대한 연구 개발이 다방면으로 진행되고 있다5-10).
본 논문에서는 수전해 기술의 특성에 대한 비교를 진행함과 동시에 International Energy Agency (IEA)에서 제공하는 자료11)를 기반으로, 대규모 수전해 실증 프로젝트의 진행 현황에 대한 기술별, 공급 전원별, 국가별, 최종 사용처별 분석을 진행하였다.
2. 수전해 기술
2.1 수전해 기술별 특성 및 성능 비교
수소 생산을 위한 다양한 수전해 기술이 존재하지만, 대표적으로는 알칼라인 수전해(alkaline water electrolysis, AWE), 고분자 전해질 수전해(polymer electrolyte membrane water electrolysis, PEMWE), 고체 산화물 수전해(solid oxide electrolysis cell, SOEC) 그리고 음이온 교환막 수전해(anion exchange membrane water electrolysis, AEMWE) 기술이 있다.
1800년 영국의 Anthony Carlisle와 William Nicholson이 수전해 기술의 개념을 확립한 이래12) AWE 기술은 약 100년의 기간 동안 연구 개발이 진행되었다. AWE 기술은 타 수전해 기술에 비해 연구 개발이 진행된 기간이 긴 만큼 높은 기술 성숙도를 가지고 있으며 다수의 상용화 및 실증 프로젝트가 진행되었고 현재도 진행 중이다13,14). PEMWE 기술의 개념은 20세기 중반부터 도입되어 연구 개발이 진행되었으며 현재 상용화 및 실증 프로젝트가 진행되고 있다15,16). PEMWE 기술은 전력 부하 변동에 대한 응답성이 뛰어나 재생전력이 연계된 그린수소 생산에 유리한 기술로 주목받고 있다. SOEC와 AEMWE 기술은 연구실 규모의 연구 개발이 활발히 진행 중이며 부분적으로 상업화 및 실증 프로젝트가 진행되고 있다.
Table 1은 각 수전해 기술별 전기화학 반응 메커니즘, 시스템 구성, 작동 조건, 특성 그리고 장단점에 대해 요약한 표이다17-20).
수전해 기술은 종합적으로 식 (1)의 반응식을 이용한다. 물(H2O)을 전기분해하기 위해 전기(e-)가 공급되면 수전해 기술별로 OH-, H+ 그리고 O2-의 이온 전도체(ion conductor)가 전해질(electrolyte)을 통과하며 수소(H2)와 산소(O2)가 발생한다. 보통 AWE, AEMWE 기술에서는 OH-, PEMWE 기술에서는 H+, SOEC 기술은 O2-를 이온 전도체로 삼는다.
(1) |
수전해를 위한 구성품은 크게 전해질(electrolyte)과 전극(electrode)으로 구분할 수 있고 수전해 기술별로 전극과 전해질에 사용되는 재료의 차이가 있다. AWE 기술은 다공성 분리막 및 액체 전해질, PEMWE과 AEMWE 기술은 고분자 전해질을 사용하며 SOEC 기술은 고체상의 세라믹 재료가 전해질로 사용된다.
AWE, AEMWE 기술에서는 내부식성을 가지고 비귀금속 촉매인 니켈(Ni)이 전극 재료로 사용된다21). 반면 PEMWE 기술에서는 전기화학 반응 촉진을 위해 귀금속 촉매인 Ir, Pt가 사용된다. SOEC 기술에서는 perovskite 구조의 La1-xSrxCoO3 (LSC), La1-xSrxCo1-yFeyO3 (LSCF), La1-xSrxMnO3-δ (LSM)이 전극 재료로 이용되고 있다22).
기술별 작동 특성의 측면에서 PEMWE, AEMWE 기술이 AWE, SOEC 기술에 비해 높은 전류밀도(to 2 A/cm2)를 가지며 SOEC 기술이 타 기술에 비해 높은 온도(600-850℃)로 구동됨을 확인할 수 있다. 또한, 수전해 시스템의 연구 개발이 지속적으로 진행되어 온 AWE, PEMWE 기술이(>50,000 hours) 아직 연구 개발 초기 단계인 SOEC, AEMWE 기술보다(> 5,000 hours) 높은 내구성을 보인다.
기술별 특성을 살펴보면, Ir, Pt 등의 귀금속 촉매가 전극 제조에 사용되는 PEMWE 기술이 타 수전해 기술에 비해 전극에 사용되는 촉매 가격이 높음을 알 수 있다. 이에 비귀금속 촉매 합성 및 Ir, Pt 촉매의 효율 향상에 관련된 연구가 다수 진행되고 있다23,24). 또한, 높은 작동 온도로 인한 단점을 가지고 있는 SOEC의 경우, 낮은 작동 온도에서의 구동과 성능 향상을 위해 proton (H+)을 이온 전도체로 사용하는 proton conducting electrolysis cell (PCEC)에 관한 연구 및 개발이 진행되고 있다25,26).
Technology readiness level (TRL)의 측면에서 AWE, PEMWE 기술은 사업화가 가능한 수준이지만, SOEC, AEMWE 기술은 시작품 단계에 그친다.
종합적으로, 기술 성숙도에 따라 현재 상용화 및 실증 프로젝트가 지속적으로 진행된 수전해 기술은 AWE, PEMWE 기술이며 SOEC, AEMWE 기술은 상대적으로 연구 개발 기간이 짧아 지속적인 투자와 연구개발로 기술의 성숙도 향상이 필요할 것으로 판단된다.
2.2 국내외 수전해 시스템 제조사별 성능 비교
Table 2는 국내외 수전해 시스템 제조사의 상용 시제품 사양을 비교한 표이다. 수전해 시스템은 단위 스택을 연결하여 전체 시스템의 용량을 구성할 수 있어 단위 스택의 용량이 크고 수소 생산에 필요한 전력량(에너지 소비량)이 낮을수록 시스템 증축 및 운용에 유리하다. 현재 대한민국 내 상용 수전해 시스템 제조사로는 (주)수소에너젠(AWE), 이엠솔루션(AWE), (주)테크윈(AWE), (주)테크로스(AWE), (주)엘켐텍(PEMWE)이 있으며, SOEC (케이세라셀), AEMWE (아크로랩스, 한화솔루션) 기술에 대한 상용화 연구 개발이 진행되고 있다6,27-31).
해외 수전해 시스템 제조사들의 공개된 자료를 바탕으로 제조사별 단위 스택 용량을 비교하였을 때, AWE 기술에서는 Sunfire사와 Asahi KASEI사가 10 MW의 용량으로 가장 큰 단위 스택을 제작하고 있으며 PEMWE 기술에서는 Cummins사의 2.5 MW 단위 스택이 가장 규모가 크다. SOEC 기술에서는 Sunfire사의 0.223 MW 단일 스택, AEMWE 기술에서는 Enapter사의 0.0024 MW 단일 스택이 가장 규모가 크다.
AWE 기술은 TRL 단계가 가장 높은 만큼 상용화 제품을 제작하는 제조사가 다른 수전해 기술에 비해 많으며, 단위 스택의 용량 또한 타 기술에 비해 평균적으로 높음을 알 수 있다. PEMWE 기술의 단위 스택 용량은 AWE 기술에 비해 평균적으로 낮지만 연구 개발이 지속적으로 이루어지고 있으며 AWE 기술 다음으로 상용화 제품 제조업체가 많고, 단위 스택의 용량 또한 SOEC, AEMWE 기술에 비해 높다. SOEC와 AEMWE 기술은 AWE, PEMWE 기술에 비해 단위 스택의 용량이 낮으며 Table 1 내용 중 TRL 단계에 비례하여 상용화 제품 제조업체의 수가 AWE, PEMWE 기술에 비해 적다. 특히, AEMWE 기술의 경우 Enapter사에서 유일하게 상용화 제품이 제조되고 있다6,32-51).
수소를 생산하기 위해 필요한 전력량(kWh)인 에너지 소모량의 측면에서 비교하면, AWE, PEMWE, AEMWE 기술은 3.8-5.0 kWh/Nm3H2의 값을 가지지만 SOEC 기술은 최소 3.3 kWh/Nm3H2의 값으로 타 기술에 비해 낮은 전력량으로 수소를 생산할 수 있어 에너지 효율이 높음을 알 수 있다.
2.3 국내외 대표 수전해 프로젝트 진행 현황 비교
전 세계적으로 탄소 감축 목표를 세부적, 단계적으로 설정하고 지속적으로 보완하며 탄소중립 실현을 위한 노력이 진행되고 있다. 2030년까지 미국은 2005년 대비 50-52%, 영국은 1990년 대비 68%, 독일은 1990년 대비 55% 탄소 배출 감축을 목표로 삼았으며 전 세계적으로 2050년까지 탄소 배출이 없는 사회를 실현하기 위해 다양한 프로젝트가 계획 및 진행되고 있다.
이와 같은 목표를 달성하기 위해 대규모 수전해 프로젝트가 다양한 국가에 의해 진행되고 있다. 그중 대표적인 수전해 프로젝트를 Table 3에 도시하였다. Table 3에서는 수전해 용량이 3 GW 이상이며 6년 이상 진행되는 프로젝트들을 선별하여 정리하였다.
수전해 기술의 선도국인 유럽에서 프로젝트를 주도적으로 진행하고 있으며 특히 스페인, 프랑스, 독일 3개국이 연계하여 2030년까지 67 GW 용량의 수전해 시스템을 제작하는 HyDeal ambition 프로젝트가 진행 중이다. 또한 H2V 프로젝트는 2030년까지 프랑스 내에 3 GW의 수전해 시스템을 증축하는 것을 목표로 삼았으며 현재 프랑스 전역에서는 목표 달성을 위해 지역별로 100-600 MW 단위의 수전해 시스템을 구축하는 세부 프로젝트가 진행되고 있다.
카자흐스탄, 오만, 모리타니 등의 국가에서는 수소를 생산하여 이를 수요처에 공급하는 것을 목표로 프로젝트를 계획 및 진행 중이다.
기업별 현황을 보면 thyssenkrupp사에서 사우디아라비아와 공급 계약을 맺어 2026년까지 NEOM City에 2 GW의 AWE 시스템을 설치 및 운전 예정이며52) Plug Power사에서는 H2 Energy Europe사와 공급 계약을 맺어 덴마크에 1 GW의 PEMWE 시스템을 공급할 예정이다53).
이와 같이 탄소중립을 달성하기 위한 대규모 수전해 실증 프로젝트가 장기적으로 계획 및 진행되고 있으며 단기적인 수전해 실증 프로젝트 또한 다수 진행 중이다54-64).
현재 국내에서 진행 중인 수전해 실증 프로젝트는 Table 4에서 확인할 수 있다. 국내에서 진행 중인 가장 큰 규모의 프로젝트는 제주에서 진행되는 ‘12.5 MW급 재생에너지 연계 대규모 그린수소 실증 기술 개발’ 과제이며 해당 과제는 AWE, PEMWE, SOEC, AEMWE 기술 총 4가지의 수전해 기술을 모두 사용하는 것이 특징이다. 제주에서 진행 중인 또 다른 프로젝트인 ‘재생에너지 연계 그린수소 생산 기술을 활용한 수소(600 kg) 및 배터리(2 MWh) 저장 시스템 기술개발 및 실증’ 과제는 3.3 MW의 규모로 2023년 현재 마무리 단계에 있다. 영광에서 진행되는 ‘그린수소 생산 시스템 신뢰성 제고 및 운영 기술개발’ 과제는 AWE, PEMWE 기술을 이용한 2.2 MW급 수전해 시스템을 구축하고 수전해 시스템 인증 센터를 운용하는 것이 목표이다65-72).
3. 국내외 수전해 실증 프로젝트 현황 및 비교
3.1 시기별 비교
21세기부터 진행된 수전해 실증 프로젝트의 대다수는 AWE, PEMWE 기술이며 2010년부터 2020년 이전까지 진행된 AWE, PEMWE 기술의 수전해 프로젝트의 연간 설치 용량을 Fig. 1을 통해 확인할 수 있다.
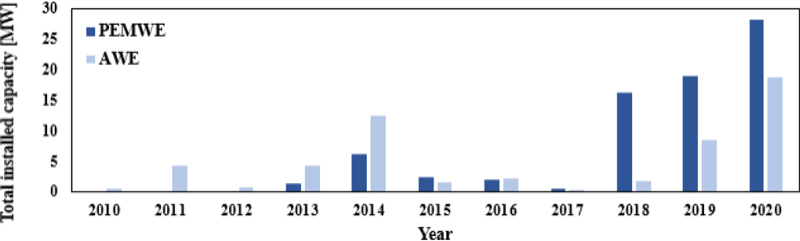
Comparison of annual installed capacity of water electrolysis demonstration projects by technology before 2020
AWE, PEMWE 기술에 대한 실증 프로젝트들의 연간 전체 설치 용량은 2020년까지 30 MW 미만의 규모였다. 그러나 2020년, 20 MW의 PEMWE 시스템 구축을 목표로 한 캐나다의 ‘Air Liquide Becancour’ 프로젝트, 10 MW의 AWE 시스템 구축을 목표로 한 일본의 ‘Fukushima Hydrogen Energy Research Field (FH2R)’ 프로젝트가 등장하였다. 이는 해당 기간 동안 진행된 프로젝트 중 최초로 구축된 10 MW 이상의 수전해 시스템이다.
하지만 2021년부터 10 MW 규모 PEMWE 시스템 제작을 목표로 한 독일의 ‘Refhyne Phase 1’ 프로젝트, 30 MW 규모 AWE 시스템 제작을 목표로 한 중국의 ‘Ningxia Solar Hydrogen Project, Phase 1’ 프로젝트와 120 MW 규모 AWE 시스템을 추가적으로 제작하는 ‘Ningxia Solar Hydrogen Project, Phase 2’ 프로젝트 등이 진행되며 10 MW 이상의 설치 용량을 갖는 수전해 실증 프로젝트들이 다수 진행되었다.
2020년부터 2025년까지 진행 및 계획된 수전해 프로젝트의 연간 설치 용량(Fig. 2)을 비교해 보면, 2021년에는 2020년에 설치된 용량(47 MW)에 비해 5.5배 증가한 259 MW 용량의 수전해 프로젝트가 진행되었고 2022년부터는 단독으로 100 MW 이상의 설치 용량을 가지는 수전해 실증 프로젝트가 등장하며 지속적으로 연간 설치 용량이 증가하였다. 2023년 현재, 총합 860 MW 의 수전해 실증 프로젝트들이 진행 중에 있으며 2024년부터는 연간 설치 용량이 1 GW 이상으로 증가하며 수전해 프로젝트의 연간 설치 용량이 GW 규모로 진입하게 된다.
3.2 공급 전원별 비교
재생전력으로 생산한 그린수소가 탄소중립 실현을 위한 방법으로 대두되며 수전해에 사용되는 전력의 출처가 중요한 기준이 되었다. 수전해 시스템에 공급되는 전력은 크게 두 가지로, 계통전력(grid power)과 재생전력(renewable power)으로 나눌 수 있다. 계통전력은 화석 연료를 기반으로 한 발전을 통해 안정적인 전력을 공급하는 것이 특징인 반면, 재생전력은 발전 과정에서 탄소 배출이 없어 친환경적이지만 출력 변동성으로 인한 불안정한 전력 공급이 단점이다.
Fig. 1과 Fig. 2는 공급 전원을 구분하지 않고 수전해 기술별 연간 설치 용량 추이를 확인하기 위해 도시된 그래프이다. 수전해 프로젝트의 연간 설치 용량이 급증하는 2020년부터 2025년의 기간 동안 수전해 기술과 공급 전원의 상관관계를 확인하기 위해 해당 기간 동안 진행 및 계획된 실증 프로젝트들의 설치 용량을 계통 전력, 재생 전력별로 분류하여 Fig. 3에 도시하였다.
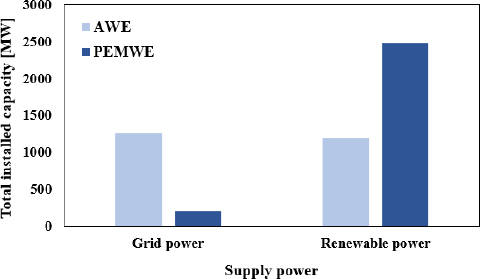
Comparison of installed capacity by supply power of water electrolysis demonstration project from 2020 to 2025
2020년부터 2025년까지의 AWE 시스템 설치 용량 총합은 2,447 MW이다. 이 중 51.2%인 1,252 MW의 설치 용량을 구성하는 프로젝트가 계통 전력을 공급 전원으로 삼았으며 나머지 48.8%인 1,195 MW의 설치 용량은 재생 전력을 공급 전원으로 삼는 프로젝트로 구성되었다. AWE 기술을 바탕으로 한 수전해 프로젝트는 공급 전원에 따라 설치 용량이 크게 차이가 나지 않음을 알 수 있다.
반면 해당 기간 동안 PEMWE 시스템의 공급 전원별 설치 용량 구성은 AWE 시스템과 구분된다. 전체 PEMWE 시스템 설치 용량인 2,683 MW 중 92.2%인 2,475 MW의 설치 용량이 재생전력을 공급 전원으로 삼는다. 해당 기간 중 PEMWE 시스템 전체 설치 용량의 92.2%가 재생전력을 공급 전원으로 삼은 것은 PEMWE 기술이 전류 변화를 추종하는 응답 속도(10%/s, Siemens Energy, München, Germany)73)가 빨라 재생전력의 변동성에 대응이 유리하여 그린수소 생산에 적합한 기술이라는 점이 영향을 미친 것으로 판단된다.
AWE 기술에서도 응답 속도(5%/s, Asahi Kasei, Tokyo, Japan)74)를 높이기 위해 차압 운전 특성 개선을 위한 시스템 개발 및 차세대 고분자 전해질막(이온솔베이팅 고분자막; Technical University of Denmark, Kongens Lyngby, Denmark)75) 개발 등이 이뤄지고 있다.
3.3 국가별 비교
Fig. 4는 2020년부터 2025년까지 진행 중이거나 진행 예정인 수전해 실증 프로젝트의 국가별 설치 용량을 공급 전원별(계통전력, 재생전력 기반)로 분류하여 도시한 그래프이다. 해당 기간 동안 수전해 프로젝트를 진행하는 주요 국가의 동향을 파악하기 위해 50 MW 미만의 설치 용량을 가진 데이터는 제외하였다.
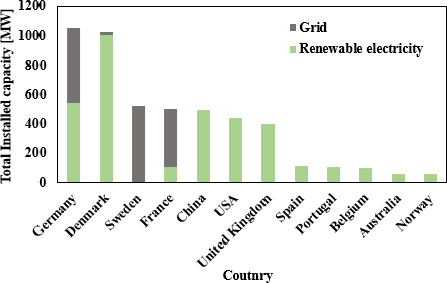
Comparison of electrolysis demonstration project capacity by country during the period from 2020 to 2025
유럽 연합은 2024년까지 6 GW의 수전해 설치 용량을 확보하는 것을 1단계, 2030년까지 40 GW의 설치 용량을 확보하는 것을 2단계 목표로 삼아 에너지 자립을 꾀하고 있다. 이를 반증하듯 위 그래프의 2020년부터 2025년까지 진행되는 모든 수전해 프로젝트의 설치 용량인 5,021 MW 중 77.3%인 3,882 MW 설치 용량이 유럽 연합에 속한 국가들이 주도하는 수전해 프로젝트이다. 독일(1,052 MW)과 덴마크(1,022 MW)는 타 국가에 비해 최소 2배 이상의 설치 용량을 가지는 수전해 프로젝트를 진행 중이거나 진행 예정이다. 대표적으로 독일 Thyssenkrupp사에서 2025년에 진행 계획 중인 500 MW 설치 용량의 ‘HydrOxy Hub Walsum’ 프로젝트가 있으며 H2 Energy사에서 덴마크의 Esbjerg 지역에 1 GW 설치 용량의 수전해 시스템을 구축하는 프로젝트가 2025년부터 진행될 예정이다.
해당 기간 동안 대부분의 국가가 재생전력을 공급 전원으로 하는 프로젝트를 진행하지만 계통전력을 함께 이용하거나(독일, 덴마크, 프랑스) 계통전력을 단독으로 공급 전원으로 사용하는 프로젝트를 진행하는 국가(스웨덴)가 있다.
3.4 최종 사용처별 비교
수전해를 통해 생산된 수소는 철강 산업, 고온용 연료, 운송 수단, 전력 생산, 합성 연료 제작 등의 다양한 분야에 사용된다. 2020년부터 2025년까지 진행되거나 진행 중인 AWE, PEMEC 수전해 프로젝트의 최종 사용처별 설치 용량을 Fig. 5에 도시하였다. 그래프에 사용한 데이터는 계통전력, 재생전력을 전력 공급원으로 삼는 프로젝트로 제한하였고, 하나의 프로젝트에서 다수의 최종 사용처에 수소를 공급하는 경우가 중복되어 포함된 경우가 있다.
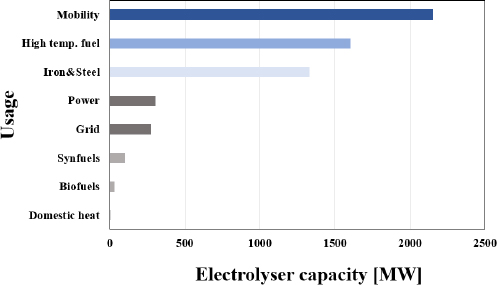
Comparison of installation capacity by end user of water electrolysis demonstration project from 2020 to 2025
2020년부터 2025년까지 진행된 계통전력, 재생전력 기반 AWE, PEMEC 수전해 프로젝트 중 생산된 수소를 운송 수단에 사용하는 프로젝트의 수전해 설치 용량(2,151 MW)이 가장 높았고, 뒤를 이어 고온의 연료가 필요한 산업(철강 산업 제외, 1,604 MW), 철강 산업(1,329 MW) 순으로 설치 용량이 높았다. 그 이후 전력 생산, 전력 계통, 합성연료 생산, 바이오연료 생산, 지역난방 순으로 설치 용량이 높았으며 생산된 수소의 대부분은 운송수단용, 고온 연료용, 철강 산업용으로 사용될 것으로 판단된다.
4. 결 론
2020년 이전 수전해 기술에 대한 실증 프로젝트의 연간 규모는 30 MW 미만이었으나 2021년 10 MW 이상, 2022년 100 MW 이상의 개별 실증 프로젝트가 등장하며 2023년 기준 총합 860 MW 설치 용량의 수전해 실증 프로젝트들이 진행 중이다. 탄소중립 사회 및 에너지 자립화 실현을 위해 전 세계적으로 수전해 실증 프로젝트의 연간 규모가 증가될 것으로 예상되며 재생전력을 이용한 그린수소 생산을 위해 앞으로 PEMWE 기술의 실증 프로젝트가 다수 진행될 것으로 판단된다. 또한 2020년부터 2025년까지 진행 중이거나 진행 예정인 수전해 시스템 설치 용량 중 77.3%가 유럽에서 진행되는 만큼, 추후 유럽이 수전해 실증 프로젝트를 가장 활발하게 진행하며 관련 산업을 주도할 것으로 판단된다.
Acknowledgments
본 연구는 산업통상자원부와 한국에너지기술평가원의 그린수소 생산 및 저장 시스템 기술개발(R&D) 사업(과제번호: 20218801010030)의 지원을 받아 수행되었습니다.
References
-
C. Wulf, P. Zapp, and A. Schreiber, “Review of power-to-X demonstration projects in Europe”, Frontiers in Energy Re-search, Vol. 8, 2020, pp. 1-12.
[https://doi.org/10.3389/fenrg.2020.00191]
-
M. Thema, F. Bauer, and M. Sterner, “Power-to-gas: electrolysis and methanation status review”, Renewable and Sustainable Energy Reviews, Vol. 112, 2019, pp. 775-787.
[https://doi.org/10.1016/j.rser.2019.06.030]
-
M. Ozturk and I. Dincer, “A comprehensive review on power-to-gas with hydrogen options for cleaner applications”, Int-ernational Journal of Hydrogen Energy, Vol. 46, No. 62, 2021, pp. 31511-31522.
[https://doi.org/10.1016/j.ijhydene.2021.07.066]
-
F. He, S. Tong, Z. Luo, H. Ding, Z. Cheng, C. Li, and Z. Qi, “Accelerating net-zero carbon emissions by electrochemical reduction of carbon dioxide”, Journal of Energy Chemistry, Vol. 79, 2023, pp. 398-409.
[https://doi.org/10.1016/j.jechem.2023.01.020]
-
A. Buttler and H. Spliethoff, “Current status of water electr-olysis for energy storage, grid balancing and sector coupling via power-to-gas and power-to-liquids: a review”, Renewa-ble and Sustainable Energy Reviews, Vol. 82, Pt. 3, 2018, pp. 2440-2454.
[https://doi.org/10.1016/j.rser.2017.09.003]
- J. Seo, S. Lee, C. Lee, J. Lee, M. Kim, S. Kim, Y. Choi, and H. Cho, “Development of water electrolysis technology for gr-een hydrogen production”, News & Information for Chem-ical Engineers, Vol. 40, No. 3, 2022, pp. 254-270. Retrieved from https://kiss.kstudy.com/Detail/Ar?key=3949657, .
- International Renewable Energy Agency (IRENA), “Green hydrogen cost reduction: scaling up electrolysers to meet the 1.5℃ climate goal”, IRENA, 2020. Retrieved from https://www.irena.org/-/media/Files/IRENA /Agency/Publication/2020/Dec/IRENA_Green_hydrogen_cost_2020.pdf, .
-
K. Roh, Y. Kim, H. Jeon, W. Kim, H. Ko, K. S. Kang, and S. U. Jeong, “Analyses on techno-economic aspects and green hydrogen production capability of MW-scale low-temper-ature water electrolyzers in Jeju Island, South Korea”, Jour-nal of Hydrogen and New Energy, Vol. 34, No. 3, 2023, pp. 235-245.
[https://doi.org/10.7316/JHNE.2023.34.3.235]
-
H. Hwang, Y. Lee, N. Kwon, S. Kim, Y. Yoo, and H. Lee, “Ec-onomic feasibility analysis of an overseas green hydrogen supply chain”, Journal of Hydrogen and New Energy, Vol. 33, No. 6, 2022, pp. 616-622.
[https://doi.org/10.7316/KHNES.2022.33.6.616]
-
S. Y. Jang, C. H. Ryu, and G. J. Hwang, “High temperature characteristics of commercially available anion exchange membrane for alkaline water electrolysis”, Journal of Hydr-ogen and New Energy, Vol. 33, No. 4, 2022, pp. 330-336.
[https://doi.org/10.7316/KHNES.2022.33.4.330]
- International Energy Agency (IEA), “World energy outlook 2022 free dataset”, IEA, 2023. Retrieved from https://www.iea.org/data-and-statistics/data-product /world-energy-outlook-2022-extended-dataset, .
-
E. Katz, “Electrochemical contributions: William Nicholson (1753-1815)”, Electrochemical Science Advances, Vol. 1, No. 2021, pp. e2160003.
[https://doi.org/10.1002/elsa.202160003]
-
A. L. Santos, M. J. Cebola, and D. M. F. Santos, “Towards the hydrogen economy-a review of the parameters that influen-ce the efficiency of alkaline water electrolyzers”, Energies, Vol. 14, No. 11, 2021, pp. 3193.
[https://doi.org/10.3390/en14113193]
-
J. Brauns and T. Turek, “Alkaline water electrolysis powered by renewable energy: a review”, Processes, Vol. 8, No. 2, 2020, pp. 248.
[https://doi.org/10.3390/pr8020248]
-
K. E. Ayers, E. B. Anderson, C. Capuano, B. Carter, L. Dalton, G. Hanlon, J. Manco, and M. Niedzwiecki, “Research adva-nces towards low cost, high efficiency PEM electrolysis”, ECS Transactions, Vol. 33, No. 1, 2010, pp. 3.
[https://doi.org/10.1149/1.3484496]
-
M. N. I. Salehmin, T. Husaini, J. Goh, and A. B. Sulong, “High-pressure PEM water electrolyser: a review on challenges and mitigation strategies towards green and low-cost hydrogen production”, Energy Conversion and Management, Vol. 268, 2022, pp. 115985.
[https://doi.org/10.1016/j.enconman.2022.115985]
-
S. S. Kumar and H. Lim, “An overview of water electrolysis technologies for green hydrogen production”, Energy Rep-orts, Vol. 8, 2022, pp. 13793-13813.
[https://doi.org/10.1016/j.egyr.2022.10.127]
- E. Zoulias, E. Varkaraki, N. Lymberopoulos, C. N. Christodoulou, and G. N. Karagiorgis, “A review on water electrolysis”, Turkish Journal of Science and Technology, Vol. 4, No. 2, 2004, pp. 41-71. Retrieved from http://www.cres.gr/kape/publications/papers/dimosieyseis /ydrogen/A%20REVIEW%20ON%20WATER%20ELECTROLYSIS.pdf, .
-
A. P. R. A. Ferreira, R. C. P. Oliveira, M. M. Mateus, and D. M. F. Santos, “A review of the use of electrolytic cells for en-ergy and environmental applications”, Energies, Vol. 16, No. 4, 2023, pp. 1593.
[https://doi.org/10.3390/en16041593]
- International Renewable Energy Agency (IRENA), “Global renewables outlook: energy transformation 2050”, IRENA, 2020. Retrieved from https://www.irena.org/publications/2020/Apr/Global-Renewables-Outlook-2020, .
-
K. Zeng and D. Zhang, “Evaluating the effect of surface mo-difications on Ni based electrodes for alkaline water electro-lysis”, Fuel, Vol. 116, 2014, pp. 692-698.
[https://doi.org/10.1016/j.fuel.2013.08.070]
-
I. D. Unachukwu, V. Vibhu, J. Uecker, I. C. Vinke, R. A. Eic-hel, and L. G. J. (Bert) de Haart, “Comparison of the electr-ochemical and degradation behaviour of Ni-YSZ and Ni-GDC electrodes under steam, Co- and CO2 electrolysis”, ECS Transactions, Vol. 111, No. 6, 2023, pp. 1445.
[https://doi.org/10.1149/11106.1445ecst]
-
L. A. King, M. A. Hubert, C. Capuano, J. Manco, N. Danilovic, E. Valle, T. R. Hellstern, K. Ayers, and T. F. Jaramillo, “A non-precious metal hydrogen catalyst in a commercial polymer electrolyte membrane electrolyser”, Nature Nanotechnology, Vol. 14, 2019, pp. 1071-1074.
[https://doi.org/10.1038/s41565-019-0550-7]
-
A. A. H. Tajuddin, G. Elumalai, Z. Xi, K. Hu, S. Jeong, K. Nagasawa, J. Fujita, Y. Sone, and Y. Ito, “Corrosion-resistant non-noble metal electrodes for PEM-type water electrolyzer”, In-ternational Journal of Hydrogen Energy, Vol. 46, No. 78, 2021, pp. 38603-38611.
[https://doi.org/10.1016/j.ijhydene.2021.09.116]
-
D. Kim, K. T. Bae, K. J. Kim, H. N. Im, S. Jang, S. Oh, S. W. Lee, T. H. Shin, and K. T. Lee, “High-performance protonic ceramic electrochemical cells”, ACS Energy Letters, Vol. 7, No. 7, 2022, pp. 2393-2400.
[https://doi.org/10.1021/acsenergylett.2c01370]
-
M. Choi, C. B. Lee, J. Baek, S. Bang, K. Hong, J. Hong, K. Kim, and W. Lee, “Engineering the surface basicity of heterogeneous catalyst for high Co2 methanation of protonic ceramic electrolysis cells”, SSRN, 2023.
[https://doi.org/10.2139/ssrn.4364666]
- E. Yoon, “Localization of water electrolysis technology... pr-omoting carbon neutrality and green growth”, Watermaeil, 2023. Retrieved from http://www.watermaeil.com/news/articleView.html?idxno=7147, .
- J. Lee, “Establish a commercial hydro electrolysis hydrogen production base”, Monthly Hydrogen Economy, 2022. Ret-rieved from https://www.h2news.kr/news/articleView.html?idxno=10066, .
- H. Kim, “Kceracell, going to localize high-temperature wat-er electrolysis technology”, igas Journal, 2021. Retrieved from http://m.igasnet.com/news/articleView.html?idxno=18034, .
- J. Lee, “<Continuous Article> Hydrogen economy, techno-logy and products attracting attention - ② ‘PEM water ele-ctrolysis technology’ of AcroLabs”, Monthly Hydrogen Ec-onomy, 2019. Retrieved from http://www.h2news.kr/news/articleView.html?idxno=7330, .
- J. Sung, “[Hydrogen industry NEW TREND] ① Develop-ment of ammonia, water electrolysis, and liquefied hydrogen technology ‘fightening’”, Monthly Hydrogen Economy, 2023. Retrieved from http://www.h2news.kr/news/articleView.html?idxno=11360, .
- Sunfire, “Hydrogen: the renewable feedstock and energy carrier”, Sunfire, 2023. Retrieved from https://www.sunfire.de/en/hydrogen, .
- Aqualyzer, “Aqualyzer: Large-scale alkaline water electroly-zer for producing hydrogen from renewable energy”, Aqua-lyzer, 2023. Retrieved from https://ak-green-solution.com/en/, .
- Verde Hydrogen, “Verde Hydrogen containerized electrolyzer solution”, Verde Hydrogen, 2023. Retrieved from https://verdehydrogen.com/verde-100-electrolyser.html, .
- thyssenkrupp nucera, “Green hydrogen solutions”, thyssenkrupp nucera, 2024. Retrieved from https://thyssenkrupp-nucera.com/green-hydrogen-solutions/, .
- John Cockerill, “Electrolysers: John Cockerill Hydrogen’s pressurized electrolyser solutions”, John Cockerill, 2022. Retrieved from https://hydrogen.johncockerill.com/en/products/electrolysers/, .
- Nel, “Atmospheric alkaline electrolyser”, Nel, 2023. Retrieved from https://nelhydrogen.com/product/atmospheric-alkaline-electrolyser-a-series/, .
- Azo Materials, “Alkaline hydrogen generators: 103 to 4,000 Nm³/h”, Azo Materials, 2023. Retrieved from https://www.azom.com/equipment-details.aspx?EquipID=7488, .
- McPhy, “McLyzer range: 200 to 3200 Nm3/h at 30 bar”, McPhy, 2023. Retrieved from https://mcphy.com/en/equipment-services/electrolyzers/large/?cn-reloaded=1, .
- H Green Hydrogen Systems, “Hyprovide® X-series”, H Gr-een Hydrogen Systems, 2023. Retrieved from https://www.greenhydrogensystems.com/electrolysers/hyprovide-x-series-6mw-modular-electrolyser, .
- Cummins, “Hydrogen: the next generation”, Cummins, 2021. Retrieved from https://www.cummins.com/sites/default/files /2021-08/cummins-hydrogen-generation-brochure-20210603.pdf, .
- Nel, “PEM electrolyser”, Nel, 2023. Retrieved from https://nelhydrogen.com/product/m-series-electrolyser/, .
- Plug, “Generate green hydrogen through PEM water electr-olysis”, Plug, 2023. Retrieved from https://www.plugpower.com/hydrogen/electrolyzer-hydrogen/, .
- H-TEC Systems, “H-TEC PEM electrolyzer modular hydr-ogen platform”, H-TEC Systems, 2023. Retrieved from https://www.h-tec.com/en/products/detail/mhp-series/mhp-product, .
- Siemens Energy, “Green hydrogen production: discover our electrolyzer and large-scale hydrogen solutions for sustain-able energy systems”, Siemens Energy, 2024. Retrieved from https://www.siemens-energy.com/global/en/home/products-services/product-offerings/hydrogen-solutions.html, .
- Elogen, “Turnkey containerised electrolysers”, Elogen, 2021. Retrieved from https://elogenh2.com/en/our-products/electrolyseurs-containerises/, .
- ITM Power, “Poseidon”, ITM Power, 2023. Retrieved from https://itm-power.com/products/poseidon, .
- Sunfire, “Hydrogen: the renewable feedstock and energy carrier”, Sunfire, 2023. Retrieved from https://www.sunfire.de/en/hydrogen, .
- Bloom Energy, “The world’s largest and most efficient solid oxide electrolyzer”, Bloom Energy, 2021. Retrieved from https://www.bloomenergy.com/bloomelectrolyzer/, .
- K. Ross, “Ceres Power to trial solid oxide electrolysis tech with Linde and Bosch”, Power Engineering International, 2023. Retrieved from https://www.powerengineeringint.com/world-regions/europe/ ceres-power-to-trial-solid-oxide-electrolysis-tech-with-linde-and-bosch/, .
- Enapter, “AEM electrolyser EL 4: jumpstart your green hy-drogen production”, Enapter, 2023. Retrieved from https://www.enapter.com/aem-electrolysers/aem-electrolyser-el-4, .
- thyssenkrupp, “One of the largest green hydrogen projects in the world: thyssenkrupp signs contract to install over 2GW electrolysis plant for Air Products in NEOM”, thyssenkru-pp, 2021. Retrieved from https://www.thyssenkrupp.com/en/newsroom/press-releases/pressdetailpage/ one-of-the-largest-green-hydrogen-projects-in-the-world%E2%80%94 thyssenkrupp-signs-contract-to-install-over-2gw-electrolysis-plant-for-air-products-in-neom-124584, .
- Plug Power, “Plug Lands 1 GW electrolyzer order with H2 energy Europe”, Plug Power, 2022. Retrieved from https://www.ir.plugpower.com/press-releases/news-details/2022/ Plug-Lands-1-GW-Electrolyzer-Order-with-H2-Energy-Europe/default.aspx, .
- HyDeal, “HyDeal ambition”, HyDeal, 2020. Retrieved from https://www.hydeal.com/hydeal-ambition, .
- J. Lillis, “Kazakhstan: oil-rich west to become green hydrogen hub”, Eurasianet, 2022. Retrieved from https://eurasianet.org/ kazakhstan-oil-rich-west-to-become-green-hydrogen-hub, .
- L. Paddison, “Oman plans to build world’s largest green hy-drogen plant”, The Guardian, 2021. Retrieved from https://www.theguardian.com/world/2021/ may/27/oman-plans-to-build-worlds-largest-green-hydrogen-plant, .
- P. Wanjala, “Top 5 low carbon energy projects in Mauritania, ongoing and incoming”, Africa Energy Portal, 2022. Retrie-ved from https://africa-energy-portal.org/news/top-5-low-carbon-energy-projects-mauritania-ongoing-and-incoming, .
- Chariot, “Green hydrogen”, Chariot, 2023. Retrieved from https://chariotenergygroup.com/operations/green-hydrogen/, .
- HyResource, “Desert Bloom Hydrogen (archived)”, HyRe-source, 2024. Retrieved from https://research.csiro.au/hyresource/desert-bloom-hydrogen/, .
- S. Matalucci, “The hydrogen stream: Europe’s largest green hydrogen project takes shape”, pv magazine, 2022. Retrieved from https://www.pv-magazine.com/2022/02/18/ the-hydrogen-stream-europes-largest-green-hydrogen-project-takes-shape/, .
- RWE, “NortH2: a green hydrogen hub in Northwest Europe”, RWE, 2020. Retrieved from https://www.rwe.com/en/research-and-development/hydrogen-projects/north2/, .
- equinor, “Equinor joins Europe’s biggest green hydrogen project, the NortH2-project”, equinor, 2020. Retrieved from https://www.equinor.com/news/archive/20201207-hydrogen-project-north2, .
- Hydrogen Island, “Hydrogen Island: an island in the North Sea dedicated to green hydrogen production”, Hydrogen Island, 2022. Retrieved from https://hydrogenisland.dk/en, .
- H2V, “H2V is developing gigafactories for the production of green hydrogen in France and Europe”, H2V, 2023. Retrieved from https://h2v.net/, .
- M. Boo, “Jeju Energy Corporation passes 3.3 MW green hydrogen production plant completion inspection and best safety management for operation of production facilities”, Jeju Energy Corporation, 2023. Retrieved from https://www.jejuenergy.or.kr/index.php/contents/notify/press?act=view&seq=2899, .
- Jeonnam Technopark, “Jeonnam Technopark selects a pu-blic offering project to build a Yeonggwang Green Hydrogen Performance Test Center”, Korea Technopark Association, 2021. Retrieved from http://www.technopark.kr/newsnissue/150510, .
- H. Kim, “Laying the foundation for the ‘building of a green hydrogen production system’ in Yeonggwang-gun”, Pressi-an, 2021. Retrieved from https://www.pressian.com/pages/articles/2021120716120955403, .
- S. Park, “Production demonstration of 12.5 MW class green hydrogen production in Jeju Island is launched in earnest”, Monthly Hydrogen Economy, 2022. Retrieved from http://www.h2news.kr/news/articleView.html?idxno=10341, .
- B. Yoon, “Korea's first commercialized water electrolysis pr-oject launched in earnest... operated in May 2025”, Electi-mes, 2023. Retrieved from https://www.electimes.com/news/articleView.html?idxno=320475, .
- S. Park, “Green hydrogen production base will be built in Pyeongchang, Gangwon Province”, Monthly Hydrogen Ec-onomy, 2022. Retrieved from http://www.h2news.kr/news/articleView.html?idxno=10278, .
- I. Jeon, “The Ministry of Commerce, Industry and Energy selected the Donghae City Consortium for the project to build a clean hydrogen production base”, Daily Kangwon-do People, 2023. Retrived from http://www.kado.net/news/articleView.html?idxno=1201015, .
- J. Sung, “Hyundai ENG to build a ‘hydrogen production base based on water electrolysis’ in Boryeong, Chungcheongnam-do”, NewDaily Economy, 2023. Retrieved from https://biz.newdaily.co.kr/site/data/html/2023/08/29/2023082900151.html, .
- Siemens Energy, “PEM electrolyser technology: flexible, ef-ficient and scalable”, Siemens Energy, 2021. Retrieved from https://www.energyforum.in/fileadmin/user_upload/india/ media_elements/Presentations/20210714_h2_large/Siemens_Energy.pdf, .
- Y. Fujita, “Asahi Kasei’s activities for green hydrogen prod-uction”, NEDO, 2020. Retrieved from https://www.nedo.go.jp/content/100939725.pdf, .
-
M. R. Kraglund, M. Carmo, G. Schiller, S. A. Ansar, D. Aili, E. Christensen, and J. O. Jensen, “Ion-solvating membranes as a new approach towards high rate alkaline electrolyzers”, Energy & Environmental Science, Vol. 12, No. 11, 2019, pp. 3313-3318.
[https://doi.org/10.1039/C9EE00832B]